Trophic Interactions and Ecosystems Flows
Current and recent
Humans affect ecosystems across the globe, often altering nutrient cycles, trophic ecology, and ecosystem function. Coastal ecosystems are often disproportionately affected due to dense human populations near the shore (Figure 1). They are also important nursery habitat for many fish species, but overabundance of nutrients causes growth of thick algal seaweed mats. Our experimental manipulation showed that seaweed outcompetes native eelgrass, alters habitat structure and invertebrate species composition, and creates inhospitable conditions for many fish species (Deegan et al. 2002, Aquatic Conservation: Marine and Freshwater Ecosystems). Our work provided the first evidence – subsequently verified across many regions – that nutrient reduction could reestablish fish nursery habitat and restore estuarine ecosystem function.
​
Anthropogenic warming strongly influences Arctic ecosystems, particularly as thawing permafrost releases long-stored carbon, nutrients, and organisms into the environment. I used the Arctic Long-Term Ecological Research program’s stream nutrient addition experiment to provide a foundation for novel ecosystem research (Figure 2). My research on trophic interactions contributed to a broader understanding of ecosystem controls in tundra streams (Bowden et al. 2014, Ecology of Streams of the Toolik Region; Deegan et al. 1997, Canadian Journal of Fisheries and Aquatic Sciences). My work on larval fish demonstrated that high fish densities can induce top-down control of aquatic invertebrate grazers, and that indirect bottom-up effects from nutrient cycling by fish can occur where background nutrients are limited (Golden & Deegan 1998, Freshwater Biology). These results were the first to demonstrate the interaction between trophic control and nutrient status in streams. I contributed to understanding the influence of climate stochasticity on fish lifecycle production, where different life-stages benefitted depending on whether warm-dry (better for young fish) versus cool-wet (better for adult fish) environmental conditions dominated during the growing season (Deegan et al. 1999, Transactions of the American Fisheries Society).
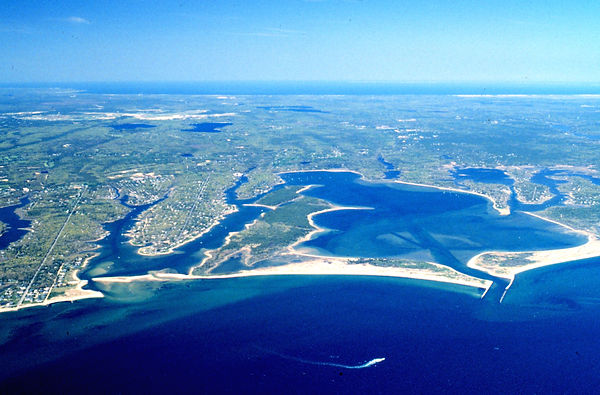
Figure 1. Waquoit Bay and neighboring estuaries on the south shore of Cape Cod, MA experience eutrophication due to excess nutrients from the surrounding urbanized watershed.
(Photo from NOAA)
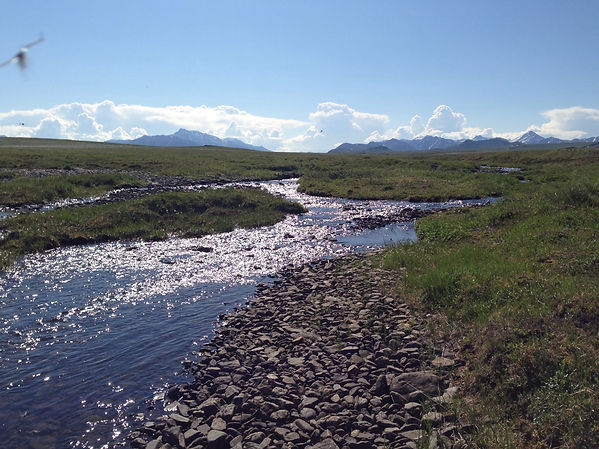
Figure 2. The Kuparuk River on the North Slope of the Brooks Mountains in the Alaskan Arctic hosts the Arctic LTER's long-term river fertilization experiment, which forms the basis for experiments testing climate change predictions. (Photo: H Golden)
My current research investigates the importance of aquatic connectivity among critical habitat patches, not only for migratory fish population persistence, but for nutrient transport among lake and stream ecosystems, as well (Figure. 3). Migratory Arctic grayling leave headwater lakes in the springtime to spawn and then forage in tundra streams throughout the summer. Our research demonstrates the dependence of headwater lake top predators (lake trout) on stream-derived nutrients from returning migrant Arctic grayling in order to maintain the high level of fish production observed within the lake.
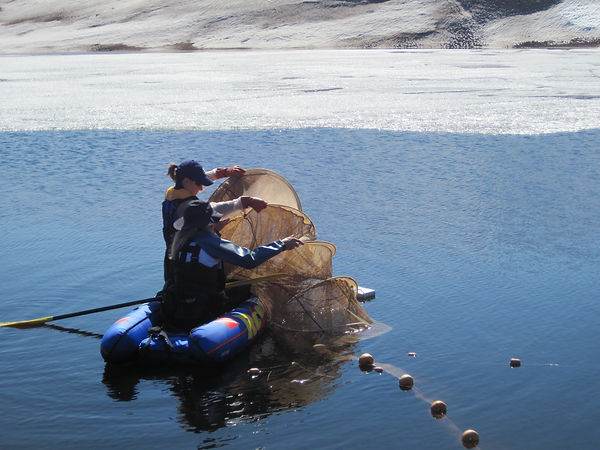
Figure 3. Sampling fish in Green Cabin Lake for stable isotopes to test predictions regarding trophic subsidy in freshwater ecosystems. (Photo: N Legere)
Future
I have begun investigating whether Arctic tundra springs might provide critical habitats necessary for long-term population resilience to changing environmental conditions. Preliminary analyses showed significant differences in aquatic macroinvertebrate species diversity among spring and non-spring reaches and among rivers with different hydrology regimes (i.e. drought-prone versus drought-resistant). Because springs do not freeze in winter and remain wet during drought these locations may provide more stable conditions that optimize ecological and environmental conditions for adult spawning, egg survival, and larval overwintering. Check out this Frontier Scientists artcle on the KUS pool.
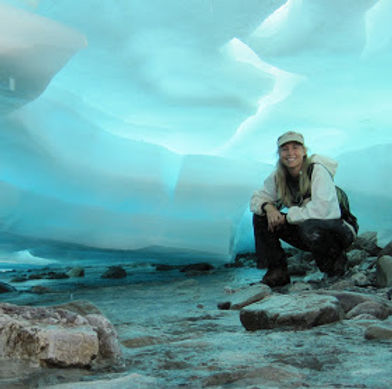
Figure 4. Arctic springs, like this one that forms the ice sheet (aufeis) near Galbraith Lake, could provide critical habitat for Arctic stream fish. (Photo: H Golden)
I am also using environmental DNA (eDNA) to investigate species distributions, monitor temporal habitat use, and assess aquatic community composition (Figure 5). In collaboration with Dr. Mark Urban
(University of Connecticut) and Dr. Linda Deegan (Woods Hole Research Center), we are developing a proposal to test hypotheses regarding metacommunity theory against climate change predictions using eDNA. Our initial samples have shown the utility of eDNA in quickly evaluating current communities, and we will extend eDNA techniques to Arctic lake sediment cores to recreate ancient fish communities in relation to past climate conditions.

Figure 5. . Filtering the icy cold water of Green Cabin Lake to collect eDNA to assess the fish community. (Photo: H Golden)
Movement Patterns, Dispersal, and Population Structure
Current and recent
Deep concern regarding rapid anthropogenic climate change in the Arctic motivated my doctoral research on aquatic habitat connectivity and population structure of Arctic fish. Arctic grayling is a freshwater migratory salmonid species that requires an interconnected network of critical habitat patches to maintain viable populations (Figure 6). My research explored whether climate-induced fragmentation of the aquatic environment might alter future Arctic grayling metapopulation structure. I assessed Arctic grayling neutral genetic structure with regard to potential drivers of genetic differentiation and found positive correlations with both stream distance and extent of river drying among populations (Golden et al. in review, Conservation Genetics, Figure 7). I also used neutral genetic analyses, passive integrated transponder (PIT) tag movement data from uniquely tagged individuals, and fecundity estimates to investigate the influence of river drying on local population vital rates, including adult mortality and repeat spawning. Further, I demonstrated that microgeographic genetic differentiation, a potential precursor to sympatric speciation, occurs among apparently well-connected Arctic grayling subpopulations (Golden et al. in prep., Canadian Journal of Fisheries and Aquatic Sciences). My results demonstrated how associations between increased habitat fragmentation, decreased genetic differentiation, and reduced population size might lead to increased extirpation risk for local populations.
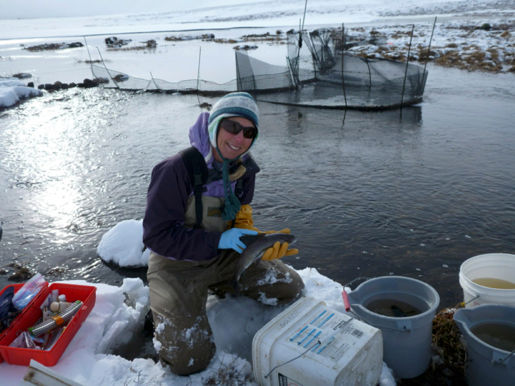

Figure 6. We genetically sampled and PIT-tagged Arctic grayling during the the open water period from early spring to early fall to assess population structure and movement patterns.
Figure 7. Our "Riverscape" analysis showed that population structure was a function of river distance and river dry zone distance among sampled locations. Bar graphs are from the program STRUCTURE, where each bar represents an individual and each color indicates individual assignment probability to five genetic clusters. Red lines approximate extent of river drying.
Urban ecosystems provide an opportunity to study rapidly occurring adaption and contribute to understanding the fundamental processes of selection. Dams along the highly populated northeastern seaboard contributed to the extirpation of many anadromous fish runs by altering aquatic connectivity and restricting access to historic spawning grounds, yet simply removing barriers does not guarantee return of historic populations. River herring populations have been in sharp declined since the late 1960s largely due to overfishing, habitat degradation, and restricted access to spawning streams by dams and road culverts (Figure 8). Working closely with the town of Falmouth, MA and a local non-profit the Coonamessett River Trust, we used PIT tags and remote PIT tag detecting arrays to monitor fish movements during the spring spawning migration. We found that herring in the Coonamessett River tend to move at night likely as a local adaptation to high daytime bird predation influenced by the removal of trees along the river for the last 100 years to facilitate farming. This river section was restored in 2018 and combined with ongoing
restoration of another river section provides opportunities to study and evaluate the effects of river and
marsh restoration on migratory fish local adaption and life-history strategies.
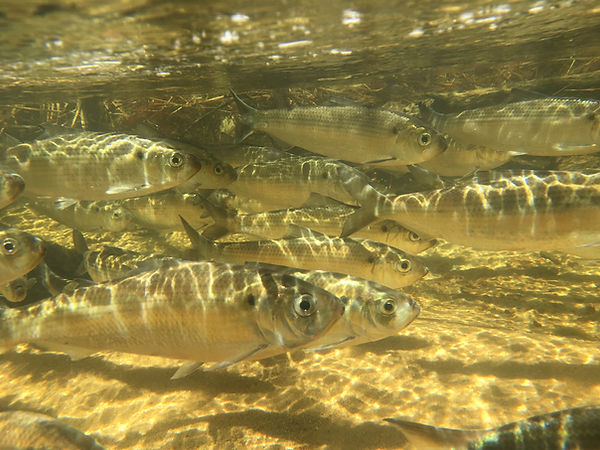
Figure 8. These river herring on the Coonamessett river expend precious energy resources waiting for flow conditions to improve before making a dash through the John Parker Road culvert en route to Flax Pond.
Future
I am exploring other techniques for investigating movement patterns, dispersal, and life-historystrategies of fish, including otolith microchemistry. In collaboration with Dr. Simon Thorrold at the Woods Hole Oceanographic Institute, I am using state-of-the-art inductively coupled plasma mass spectrometry (ICP-MS) to analyze isotopic ratios comprising the crystalline rings within the ear bones (otoliths) of fish. Fish otoliths incorporate and retain chemical signals from the water in which the fish reside. As such, the chemical signal in adult Arctic grayling otolith cores represents water chemistry from the time and location of first-year growth. I intend to use otolith microchemistry to examine differences in movement patterns among river systems and to estimate the location of critical habitats, such as spawning sites and overwintering locations. I predict that otolith core chemistry will correlate with geographically distinct tundra springs, suggesting that these locations provide critical habitat for first-year survival.Additionally, strong site fidelity to spring locations, determined using otolith microchemistry, might provide a rationale for microgeographic genetic differentiation observed within apparently well-connected populations. Similar to tree rings, fish otoliths also hold individual age and growth information.
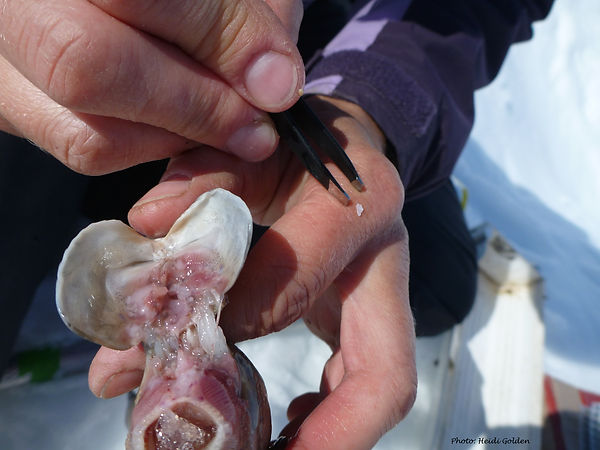
Figure 9. Tiny fish ear bones function as biological hard drives, storing information that can be used to determine growth, movement, and life-history patterns unique to each individual.
Selection, Life-History Strategies, and Eco-Evolutionary Feedbacks
​
Current and recent
To understand potential population resilience to climate change, I investigated local adaptation among fish populations from watersheds with different temperature and hydrology profiles using common garden and reciprocal transplant experiments. Common garden experiments are challenging under the best conditions and creating the infrastructure for our aquatic common garden at our remote field station on Alaska’s North Slope was no exception! We used families of individuals created by capturing and strip-spawning adult fish from multiple populations of Arctic grayling (Figure 10). Our common garden experiment showed that fish from our cooler watershed grew faster and had higher metabolic rates than fish from our warmer watershed, particularly when raised under predicted climate
change conditions. However, the trade-off for increased growth came with reduced survival. We are still analyzing samples from our reciprocal transplant experiment, a potential benefit for interested student scientists, and predict similar results from this field-based investigation to those obtained in the laboratory. Results from this research
provide insight regarding local population susceptibility to extirpation due to climate change.

Figure 10. Stripping eggs from a female Arctic grayling from the upper Kuparuk River population, which were then fertilized and used in our common garden and transplant experiments. And, surprise! This fish's PIT-tag was also stripped along with her eggs!
Future
Combining insights from years of research provides a framework for generating hypotheses regarding potential drivers for the evolution of life-history strategies. For example, site fidelity to critical habitat locations that increase survivorship might influence neutral genetic structure and might also create strong selection for life-history strategies within a population, thereby facilitating selectively reinforced trophic subsidy among connected ecosystems. I am continuing my research on selection, life-history strategies and eco-evolutionary feedbacks by testing for genomic signs of selection with Arctic grayling using restriction enzyme associated DNA sequencing (RADseq). I am now discovering areas of the genome that are under local selection and from this I will infer protein associations using the well-studied and closely related Atlantic salmon genome as a genetic template. This genomics research is conducted at the University of Connecticut with the Center for Genome Innovation, including cutting-edge next generation sequencing with Dr. Bo Reese and Dr. Kendra Maas and bioinformatics with Dr. Jill Wegrzyn.
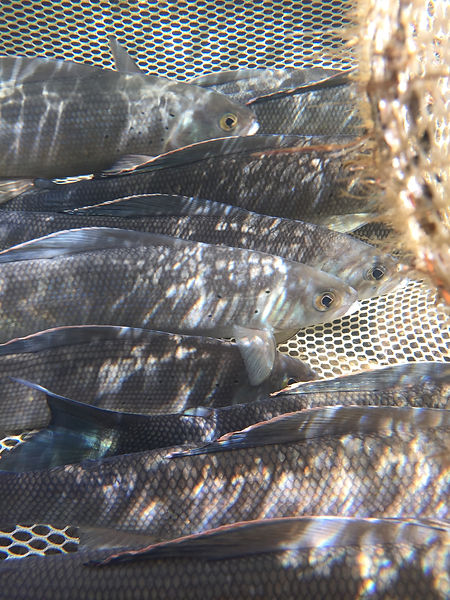
Figure 11. Genomic research from Arctic grayling populations, like these fish from the lower Oksrukuyik Creek, might help us to better understand ecology, evolution and eco-evolutionary feedbacks in a rapidly changing world.